Accumulation flows that occur when gas surrounds massive celestial bodies are a common phenomenon in the vast expanses of the universe. The light emitted by the accretion disk is a potential indicator of the existence of a black hole. However, the behavior of gas falling onto a black hole remains a mystery to scientists, and they often come up with theoretical explanations to unravel its mysteries.
try details
Laboratory attempts to understand the physics of accretion disks are carried out by various methods. Some physicists have modeled the phenomenon by creating flows of water-glycerine solutions or metal melts in the presence of a magnetic field. Another method involves applying an electric current to the edge of a Hall plasma held by permanent magnets. However, these methods have a significant drawback – they contain strict boundaries that do not exist in natural cosmic processes and lead to distortion of simulation results.
To overcome these limitations, a group of physicists led by Sergey Lebedev of Imperial College London, in collaboration with colleagues in the United States, designed an experiment that solves this problem. The experiment was: oblique collision of eight plasma jets, twisted intricately to form a ring. The motion observed in these plasma jets was similar to the motion of matter in the accretion disk of a massive celestial body. In addition, the experiment created characteristic plasma jets perpendicular to the spin plane.
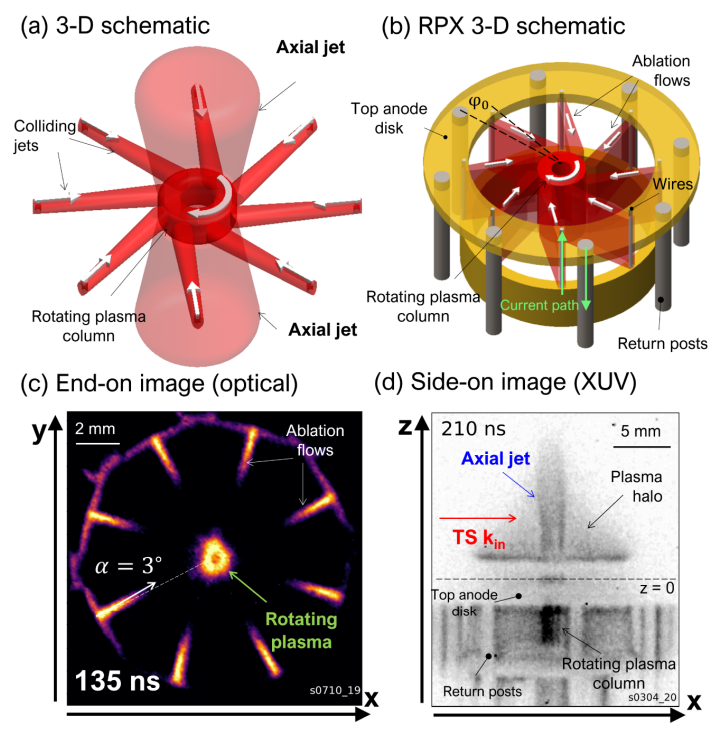
Schematic representation of plasma flows in the experiment / Photo Physical Review Letters
The setup the physicists used consisted of 40 micrometer-thick aluminum wires placed in the middle of the sides of a regular octagon. The material was heated and evaporated by passing large pulses of current through these wires, whose peak values ​​were up to 1.4 megaamps. The resulting magnetic fields directed the ablation streams to the center of the setup and deflected them slightly from the central axis.
The collision of these plasma streams caused them to coalesce into a six millimeter diameter ring. This ring existed for a short period of time, about 210 nanoseconds, during which time the plasma made one and a half to two full cycles. Physicists carefully followed the formation and evolution of the plasma ring, using optical and ultraviolet observations that allowed them to study the distribution of velocities. The resulting images showed the stability of the plasma ring during its short life and the rotation that occurred in the quasi-Keplerian regime.
In addition to the plasma ring, the researchers also observed the appearance of a plasma jet produced from a plasma column rotating under the influence of axial thermal and magnetic pressure gradients. The matter in the jet developed a speed of about 100±20 kilometers per second. A narrow declination angle of 3±1 degree indicated that there were no instability effects. The plasma jet was surrounded by a plasma halo.
Other experiments
Looking ahead, the study authors plan to extend the life of the plasma ring by using longer ablation pulses that would require thicker wires. They are trying to gain control over various parameters of magnetodynamic flow by replacing aluminum with alternative materials. This approach will shed light on the role of magnetic field instability in matter deposition, allowing them to approximate conditions found in astrophysical processes.
While the accretion disk isn’t the only phenomenon associated with black holes that physicists are trying to reproduce in laboratory experiments, this latest achievement marks an important step forward.